Introduction
At high temperatures, the radiation emitted by a plasma includes
the free-free (bremsstrahlung) and free-bound (recombination) X-ray
continuum radiation as discussed in
previous nuggets .
But it also includes thousands of very narrow
lines from the different elements in the plasma in addition to the
dominant hydrogen.
These are produced in a myriad of different ways
as the atoms in different ionization stages change from one quantum
energy state to another and emitting photons at characteristic
energies.
Fortunately, the latest version of the
CHIANTI codes and atomic data base allows the spectrum of both
the continuum and the line emission to be calculated for a plasma
with a given temperature and an assumed set of abundances for all
elements up to iron and nickel.
Certain assumptions also have to
be made about
ionization equilibrium.
This should not be a problem during
the decay phase of a flare when any plasma is likely to be in thermal
equilibrium but may be an issue during the impulsive phase.
The elusive evidence for nonthermal equilibrium could be an exciting
side-benefit of any discrepancies found between the predicted and measured
spectra during impulsive variation.
The basic method for determining the abundances of the different
elements in the plasma is to measure the intensities of various
X-ray lines from each element during a flare.
Broadly speaking, the
intensity of a line from a particular element for a plasma with a
given emission measure and temperature is directly proportional to
the abundance of that element.
Previous X-ray spectral measurements were made using mainly
Bragg crystal spectrometers.
These cover narrow energy ranges but with
very fine energy (or wavelength) resolution so that individual lines
from iron or calcium or sulfur can be resolved.
This has allowed the line intensities to be determined very accurately.
The problem has instead been to determine the plasma temperature and emission
measure with commensurate precision.
The thermal continuum X-ray spectrum helps with both these quantities, but
it has generally been difficult to measure because of fluorescence radiation
from the Bragg-scattering crystals used in the instruments.
Instead, line ratios and other techniques have been used in the past
to estimate the required temperature and emission measure.
Despite these difficulties, a large number of abundance measurements
have been made of flaring plasma confirming the "FIP Effect."
This is dependence of the elemental abundance on its first
ionization potential (FIP).
Low-FIP elements (FIP below 10 eV) tend to have higher (factor of 2-4)
abundances in flares than in the photosphere while high-FIP elements tend
to have the same abundances as in the photosphere, or even lower.
The differences in the abundances of different elements in the corona and
photosphere offer the intriguing possiblity of being able to determine
the origin of the hot plasma that appears during flares.
If the abundances tend to be more similar to photospheric, then one
could conclude that the plasma originated from the chromosphere as
expected from the
Neupert effect,
in which chromospheric plasma is heated and "evaporates" into the corona.
If measured abundances tend to be more similar to coronal values, with
enhanced low-FIP elements, then one could conclude that the hot plasma
originated from the corona and was directly heated in situ.
Measuring such abundance differences
during a flare and for different flares would be extremely valuable
in understanding the energy release process itself.
RHESSI has contributed measurements of the iron-line complex at
~6.7 keV as discussed in a
previous nugget . These show good agreement with the CHIANTI-predicted
equivalent widths for coronal iron abundances that are four times
the photospheric value.
Further data analysis suggests complications in this conclusion, though,
so it would be nice to have help interpreting the RHESSI spectra.
The MESSENGER X-ray Spectrometer
A new instrument has recently become available for making solar
X-ray observations in the critical 1 to 10 keV energy range.
This is the X-ray Spectrometer (XRS) that makes up part of the MErcury
Surface, Space ENvironment, GEochemistry and Ranging instrument
(MESSENGER).
The
MESSENGER spacecraft was launched on August 3, 2004.
It has already flown past Mercury once and will eventually
go into orbit to study the characteristics of the planet and its
environment.
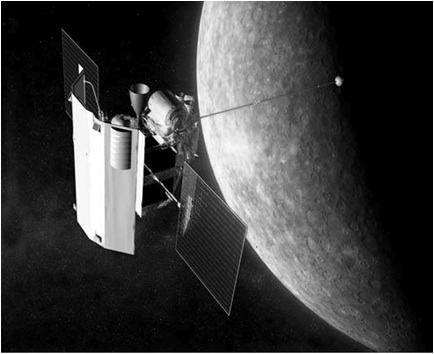
Figure 1:
Artist's impression of MESSENGER in orbit about Mercury,
showing the sunshade on which the XRS solar monitor is mounted.
The XRS consists of three gas proportional counters to detect solar-induced
fluorescence from the surface of the planet, plus a small solar monitor
mounted on the MESSENGER sunshade (Figure 1).
The solar monitor consists of a single
Si PIN detector with a sensitive area of only 0.03 mm2.
Its primary purpose is to provide the spectrum of the solar X-ray flux
incident on Mercury so that the MESSENGER measurements of the resulting
fluorescence radiation can be interpreted to provide surface composition.
Despite its small size, the XRS solar monitor is providing broad-band
measurements of the solar X-ray thermal continuum emission from 1 to 10 keV
that give accurate values for the flare plasma temperature and
emission measure.
Its energy resolution of ~0.7 keV (FWHM) is somewhat better than
RHESSI's, and can clearly resolve the line complexes of Fe at 6.7 keV and
Ca at 3.9 keV.
These abundances can therefore be estimated fairly directly.
In addition, the abundances of Si, S, and Ar can be estimated from the
increases over the continuum emission resulting from the broad complexes
of lines from these elements at energies below 3.5 keV not accessible
to RHESSI.
Precisely because the XRS detector is so small, it will not
become saturated by high counting rates or suffer from pulse pile-up
except during the peaks of the very brightest flares.
Consequently, we hope that future coincident measurements during large
flares with XRS and RHESSI will provide much needed help in interpreting
the RHESSI spectral observations below 10 keV and in normalizing the
measurements in the different attenuator states.
XRS Flare X-ray Spectra
The largest flare seen by MESSENGER since its launch occurred on 1 June 2007.
The XRS light curves are shown for four energy bands in Fig. 2.
Note that XRS accumulates spectra for 5 minute intervals.
This is a limitation for the more impulsive flares in hard X-rays but
for the thermal component below 10 keV, this is not such a serious
problem although, of course, a higher cadence would have been preferred.
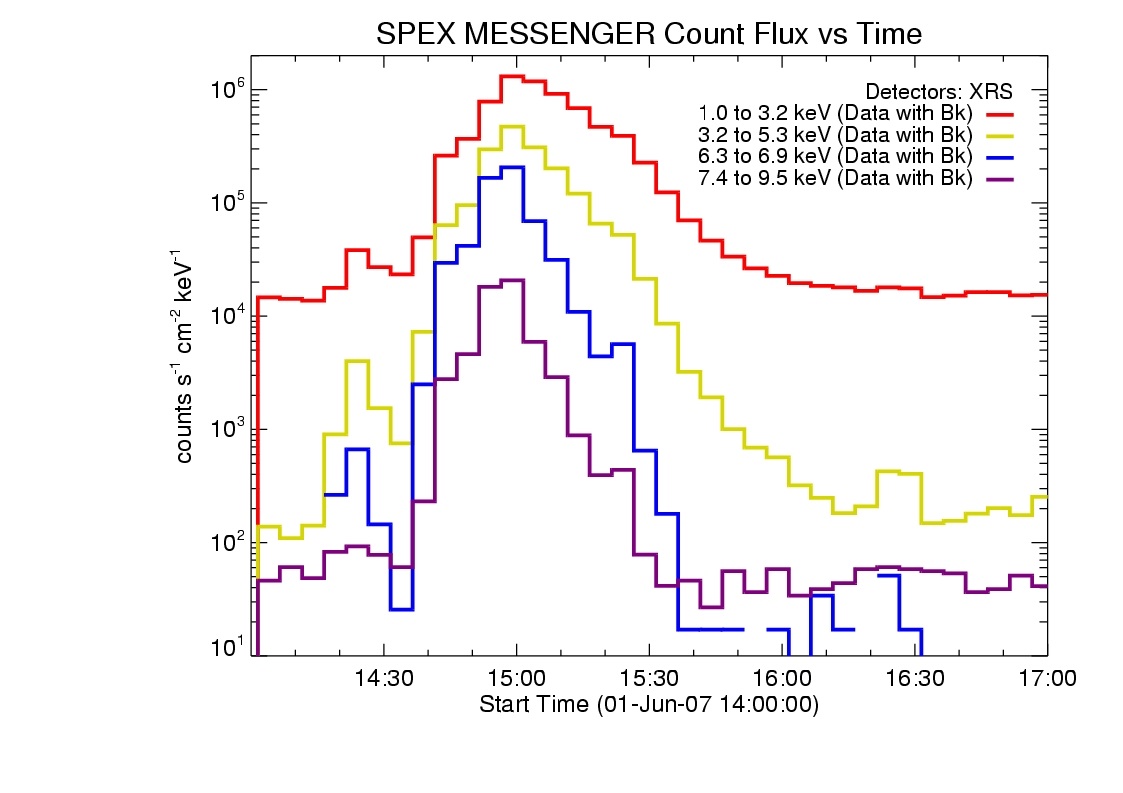
Figure 2:
X-ray light curves measured with XRS in four energy bands for
the flare starting at 14:30 UT on 1 June 2007.
Note that the lower energies show the typical later peak times and longer
decay times compared to the higher energies.
Detailed spectra extending from 1 to ~9 keV are available from XRS for each
5-min. interval during the flare.
An example is shown in Fig. 3, where the measured counting rate is
plotted versus energy at the time when the best-fit temperature of
the emitting plasma reached a peak value of about 23 MK.
Figure 4 shows the CHIANTI-predicted contributions of the line and
continuum emission from the different elements in the flare plasma to
the total count-rate spectrum measured by XRS.
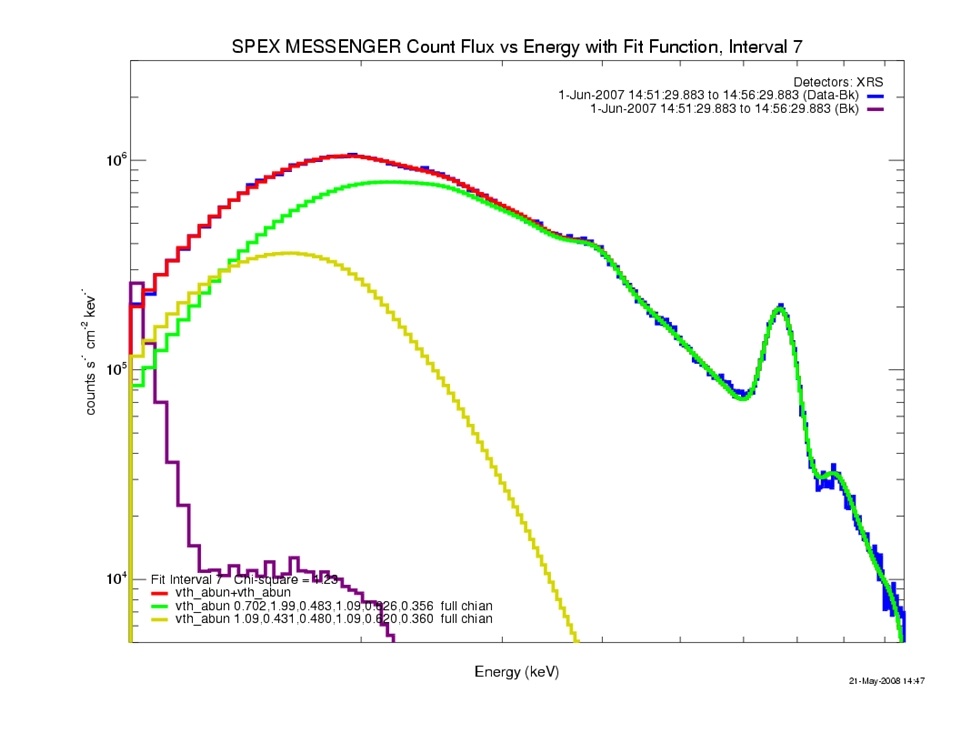
Figure 3:
MESSENGER XRS spectrum with CHIANTI thermal best-fits.
Blue histogram: background-subtracted X-ray count-rate spectrum measured
with XRS at the time of peak temperature during the flare starting at 14:30 UT
on 1 June 2007.
Green and yellow curves: CHIANTI-predicted line-plus-continuum thermal
spectra that when summed (red curve) give the best fit to the data.
Purple histogram: pre-flare backgound spectrum.
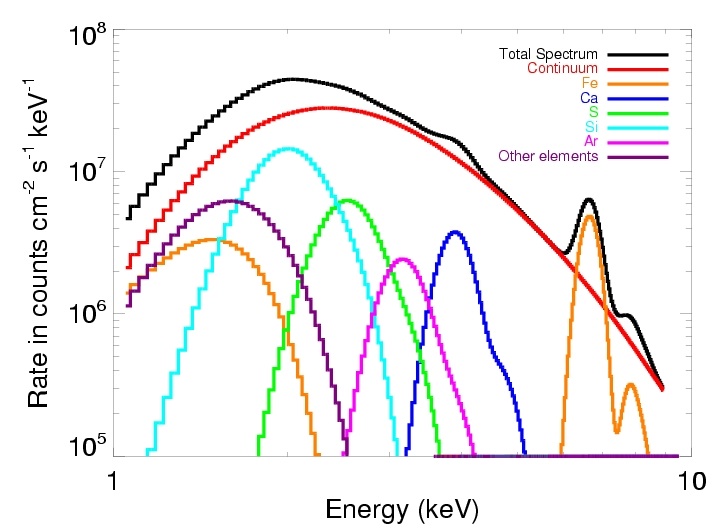
Figure 4:
Continuum and line contributions to the measured XRS count-rate spectrum.
The black curve is the best fit to the XRS count-rate spectrum and the
colored lines are the contributions from the continuum and the line
complexes from the different elements, as shown in the legend.
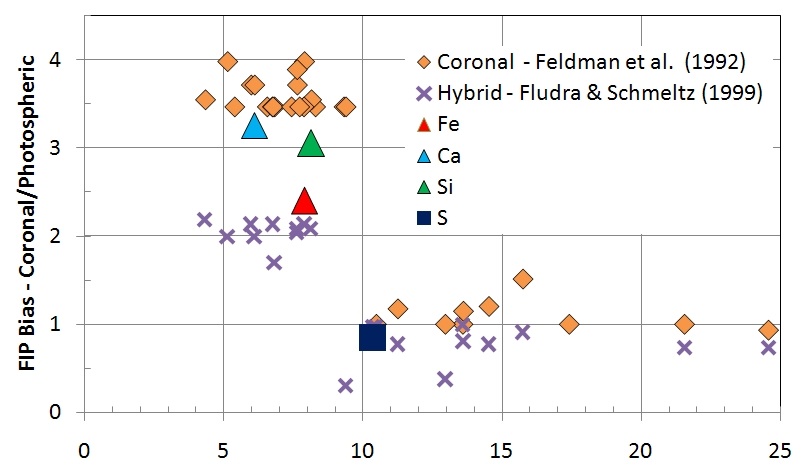
Figure 5:
FIP bias vs. FIP for two different models of coronal abundances used by
CHIANTI and for the XRS-measured values for Fe, Ca, Si, and S averaged
over five ~M-class flares.
Conclusions
The MESSENGER X-ray spectrometer is providing high-resolution measurements
of the soft X-ray emission from solar flares that can be used to determine
the elemental abundances of the heated plasma.
The preliminary results shown in Fig. 5 suggest that the relative abundances
of Fe, Ca, and Si are enhanced with respect the photospheric values but
not by as large a factor as given in the "coronal" abundancs used by CHIANTI.
The relative abundance of S is consistent with its photospheric value as
expected given its intermediate FIP of 10.4 eV.
While these early results do not allow a definitive answer to be given
concerning the origin of the flare hot plasma, they do suggest that
direct in situ heating may play a significant role even during the flare
impulsive phase and that chromospheric evaporation may not provide all of
the hot plasma to the corona during a flare.
Future measurements with XRS of larger flares should address this
question more definitively.
Biographical note:
Brian Dennis is a solar physicist at NASA's Goddard Space Flight Center,
and is the RHESSI Mission Scientist and a CoI.
Richard Starr is the MESSENGER XRS Inastrument Scientist working for
The Catholic University of America at Goddard Space Flight Center.