Evidence for a Coronal Shock Wave Origin for Relativistic Protons Producing Solar Gamma-Rays and Observed by Neutron Monitors at Earth
From RHESSI Wiki
(→Introduction) |
|||
(32 intermediate revisions not shown) | |||
Line 3: | Line 3: | ||
|title = Evidence for a Coronal Shock Wave Origin for Relativistic Protons Producing Solar Gamma-Rays and Observed by | |title = Evidence for a Coronal Shock Wave Origin for Relativistic Protons Producing Solar Gamma-Rays and Observed by | ||
Neutron Monitors at Earth | Neutron Monitors at Earth | ||
- | |number = | + | |number = 375 |
|first_author = Athanasios Kouloumvakos | |first_author = Athanasios Kouloumvakos | ||
|second_author = Gerald Share | |second_author = Gerald Share | ||
- | |publish_date = 16 | + | |publish_date = 16 April 2020 |
- | |next_nugget = | + | |next_nugget = Filament eruption |
- | |previous_nugget = {{#ask: [[Category:Nugget]] [[RHESSI Nugget Index:: | + | |previous_nugget = {{#ask: [[Category:Nugget]] [[RHESSI Nugget Index::374]]}} |
}} | }} | ||
== Introduction == | == Introduction == | ||
- | There have been several Nuggets discussing what was earlier referred to as Long Duration | + | The [https://fermi.gsfc.nasa.gov Fermi] |
- | Gamma-Ray Flares. | + | γ-ray observatory has shown that powerful solar eruptive events often emit copious fluxes of high-energy (>100 MeV) γ-rays, at times after the impulsive flare phase. These observations have become a powerful tool with which to explore the high-energy processes, such as particle acceleration, that |
- | observed by Fermi LAT, we decided that Late Phase Gamma-Ray Emission (LPGRE) best | + | constitute a major part of the eruptions' physical development. |
- | describes the characteristics of this phenomenon (Ref 1 | + | There have now been several Nuggets discussing what was earlier referred to as ''Long Duration Gamma-Ray Flares'', including |
- | emission is from | + | ([http://sprg.ssl.berkeley.edu/~tohban/wiki/index.php/Dimmings_and_Sustained_Gamma-Ray_Events No. 179], [http://sprg.ssl.berkeley.edu/~tohban/wiki/index.php/Solar_Cosmic_Rays,_Neutrons,_and_Fermi_Gamma-Rays No. 227], |
- | with a distinctly different | + | [http://sprg.ssl.berkeley.edu/~tohban/wiki/index.php/A_Lasso_Model_for_Solar_Gamma-ray_Events No. 300], |
- | time history, or up to two hours later; 3) rise times range from a few minutes to 6 hr and | + | and recently [http://sprg.ssl.berkeley.edu/~tohban/wiki/index.php/The_Temporal_and_Spatial_Extension_of_Gamma-ray_Emission_from_the_Sun No. 370], |
- | durations from 5 minutes to 20 hr; 4) there are typically ten times more >500 MeV protons | + | as well as [http://sprg.ssl.berkeley.edu/~tohban/wiki/index.php/SOL2017-09-04_(M5.5)_2017_as_a_Source_of_Relativistic_Electrons_and_Protons No. 373]) |
- | producing the LPGRE than the flare γ-ray emission; 5) 28 of the 30 LPGRE events were | + | |
- | associated with fast and broad CME's and 29 of the 30 were associated with Type ll radio | + | Based on a detailed study of thirty >100 MeV γ-ray events |
- | emission | + | observed by Fermi LAT, we decided that ''Late Phase Gamma-Ray Emission'' (LPGRE) best |
- | solar energetic particle (SEP) durations appear to be correlated and the number of >500 | + | describes the characteristics of this phenomenon (Ref [1]): |
- | MeV protons producing the LPGRE appear to range from 0.1 to 50% of the number in the | + | <ul> 1) the γ-ray emission is from [https://en.wikipedia.org/wiki/Pion pion] decays; </ul> |
- | accompanying SEP with large systematic effects; and 7) accompanying >100 keV impulsive | + | <ul> 2) it can begin close to the onset of the impulsive solar flare, but with a distinctly different time history, or up to two hours later; </ul> |
- | bremsstrahlung suggests the presence of a suprathermal flare proton seed population that | + | <ul> 3) rise times range from a few minutes to 6 hr and durations from 5 minutes to 20 hr;</ul> |
- | is accelerated by a shock. | + | <ul> 4) there are typically ten times more >500 MeV protons producing the >100 MeV LPGRE than the flare >100 MeV γ-ray emission; </ul> |
+ | <ul> 5) 28 of the 30 LPGRE events were associated with fast and broad Coronal Mass Ejections (CME's) ([https://www.swpc.noaa.gov/phenomena/coronal-mass ejections CME]) and 29 of the 30 were associated with Type ll radio emission; </ul> | ||
+ | <ul> 6) LPGRE and >100 MeV solar energetic particle (SEP) durations appear to be correlated and the number of >500 MeV protons producing the LPGRE appear to range from 0.1 to 50% of the number in the accompanying SEP with large systematic effects; and </ul> | ||
+ | <ul> 7) accompanying >100 keV impulsive bremsstrahlung suggests the presence of a suprathermal flare proton seed population that is accelerated by a [https://www.space.com/nasa-detects-first-interplanetary-shock.html shock wave] and contributes to the LPGRE.</ul> | ||
These characteristics support suggestions, beginning as early as the 1980's, that LPGRE | These characteristics support suggestions, beginning as early as the 1980's, that LPGRE | ||
- | is produced by the same CME-shock acceleration process that accelerates SEPs Ref). | + | is produced by the same CME-shock acceleration process that accelerates SEPs (Ref [2]). |
- | However, this premise is not universally accepted | + | However, this premise is not universally accepted. In this Nugget we discuss a |
- | recent study (Ref | + | recent study (Ref [3]) of the 2017 September 10 solar eruptive event that produced |
- | high-energy γ-ray emission | + | high-energy γ-ray emission having a complex time history (Ref [4]) and a ground-level |
- | enhancement (GLE) | + | enhancement ([https://en.wikipedia.org/wiki/Neutron_monitor GLE]) designated as |
- | GLE72. The γ-ray emission observed by | + | GLE72 (a numerical [http://gle.oulu.fi/#/ index]) that began with SOL1956-02-23. |
+ | |||
+ | We examine a common shock-acceleration origin for both the LPGRE and GLE72. | ||
+ | The >100 MeV γ-ray emission observed by the [https://fermi.gsfc.nasa.gov Large Area Telescope] | ||
+ | (LAT) on the ''Fermi'' satellite exhibits a weak impulsive phase, | ||
consistent with that observed in hard X-and γ-ray line flare emissions, and what | consistent with that observed in hard X-and γ-ray line flare emissions, and what | ||
appear to be two distinct stages of LPGRE. From a detailed modeling of the shock wave, we | appear to be two distinct stages of LPGRE. From a detailed modeling of the shock wave, we | ||
- | derive the 3D distribution and temporal evolution of | + | derive the 3D distribution and temporal evolution of various shock parameters, and we examine |
the shock wave magnetic connection with the visible solar disk. The evolution of shock | the shock wave magnetic connection with the visible solar disk. The evolution of shock | ||
- | parameters on field lines returning to the visible disk | + | parameters on field lines returning to the visible disk mirrors the two stages of LPGRE. |
The time history of shock parameters magnetically mapped to Earth agrees with the rates | The time history of shock parameters magnetically mapped to Earth agrees with the rates | ||
- | observed by the Fort Smith neutron monitor during the first hour of the GLE72 if we | + | observed by the |
+ | [http://neutronm.bartol.udel.edu/stations/fortsmith.html Fort Smith] neutron monitor during the first hour of the GLE72 if we | ||
include a 30% contribution of flare-accelerated protons during the first ten minutes, | include a 30% contribution of flare-accelerated protons during the first ten minutes, | ||
- | having a release time following the time history of nuclear gamma-rays. | + | having a release time following the time history of nuclear gamma-rays. |
==Comparison of Gamma-Ray Observations with Results of Shock Modeling== | ==Comparison of Gamma-Ray Observations with Results of Shock Modeling== | ||
- | The black data points in Figure 1 | + | The black data points in Figure 1 show the time history of the |
>100 MeV γ-ray fluxes over the whole event and for the first 30 minutes (inset). | >100 MeV γ-ray fluxes over the whole event and for the first 30 minutes (inset). | ||
- | [[File: | + | [[File:375f12.png|700px|thumb|center|'''Figures 1 and 2''': Left, Comparison of the >100 MeV |
- | + | γ-ray time history with an empirical shock model for different critical Mach numbers. | |
- | + | The inset shows a blowup covering the first orbit of LAT observations. | |
+ | Right, Time histories of a) 100-300 keV emission, b) amplitude of >300 keV bremsstrahlung observed by GBM, | ||
+ | c) nuclear deexcitation line emisson observed by GBM, and | ||
+ | d) >100 MeV γ-rays during the first 15 minutes of the event.]] | ||
We compare the | We compare the | ||
- | time history of this high-energy emission for the first 15 | + | time history of this high-energy emission for the first 15 minutes with lower energy |
- | emissions in Figure 2 | + | emissions in Figure 2. The 100-300 keV (RHESSI) plot in panel a) shows the impulsive |
- | flare bremsstrahlung that extends to >1 MeV based on fits to the Fermi/GBM (panel b) | + | flare bremsstrahlung that extends to >1 MeV based on fits to the Fermi/GBM |
- | + | spectra (panel b). These fits also reveal the presence of nuclear de-excitation γ-ray lines produced by | |
flare-accelerated ions (panel c). There is evidence for this flare component in the first | flare-accelerated ions (panel c). There is evidence for this flare component in the first | ||
minutes of >100 MeV γ-ray emission before the rapid rise of the first stage of LPGRE at | minutes of >100 MeV γ-ray emission before the rapid rise of the first stage of LPGRE at | ||
~15:58 UT (panel d). The second stage of LPGRE becomes evident after 16:05 UT. | ~15:58 UT (panel d). The second stage of LPGRE becomes evident after 16:05 UT. | ||
Observations at later times indicate that there was a striking decrease in the flux | Observations at later times indicate that there was a striking decrease in the flux | ||
- | between 16:30 and 18 UT | + | between 16:30 and 18:00 UT. |
- | + | ||
- | + | ||
We set out to determine whether what appear to be at least two stages of LPGRE can be | We set out to determine whether what appear to be at least two stages of LPGRE can be | ||
explained by CME related shock acceleration of protons onto magnetic field lines | explained by CME related shock acceleration of protons onto magnetic field lines | ||
returning to the visible solar disk. This study follows the CME mapping and shock | returning to the visible solar disk. This study follows the CME mapping and shock | ||
- | parameter procedures adapted in | + | parameter procedures adapted in (Ref [5]). We used 3D modelling of the CME |
- | expansion into the corona and interplanetery space to model | + | expansion into the corona and interplanetery space to model various shock parameters |
- | with time. In Figure 3a | + | with time. In Figure 3a we plot the density compression ratio |
- | along the shock front at 16 UT. This ratio is a measure of the efficiency for particle | + | along the shock front at 16:00 UT. This ratio is a measure of the efficiency for particle |
acceleration. In Fig 3b we plot representative field lines connected to the shock front, | acceleration. In Fig 3b we plot representative field lines connected to the shock front, | ||
color coded with the density compression ratio. In Fig 3c we plot the ratio as projected | color coded with the density compression ratio. In Fig 3c we plot the ratio as projected | ||
- | onto the solar disk by these field lines. At this time, most of particle acceleration | + | onto the solar disk by these field lines. At this time, the highest ratios, and therefore most of the particle acceleration, |
- | appears to occur on closed field lines emanating from the active forming an ellipse | + | appears to occur on closed field lines emanating from the active region with distant footpoints forming an ellipse. The pink shaded region is not |
- | + | ||
visible from Earth. Similar plots can be made for Alfvenic Mach number and magnetic field | visible from Earth. Similar plots can be made for Alfvenic Mach number and magnetic field | ||
direction relative to the shock normal. | direction relative to the shock normal. | ||
+ | [[File:375f34.png|700px|thumb|center|'''Figures 3 and 4''': Left, a) distribution of density compression ratios on the CME shock surface at 16:00 UT, b) magnetic field lines connected to the shock front color coded by the density compression ratio, c) Carrington map of density compression ratios projected onto the solar disk by the field lines. | ||
+ | Right, Comparison between the temporal evolution of an empirical shock parameter on field lines reaching Earth for different particle delays with the time history of count rates from the Fort Smith neutron | ||
+ | monitor. | ||
+ | Red curve shows best fitting contributions of an impulsive flare component and the shock parameter for a particle transport time of 12.5 minutes.. | ||
+ | ]] | ||
Using this technique, we made maps of shock parameters on the visible solar disk as | Using this technique, we made maps of shock parameters on the visible solar disk as | ||
- | + | functions of time to construct their temporal evolution. In order to more accurately | |
- | + | estimate the proton impact at the Sun, we took into account the probability that the | |
- | particles could reach the Sun in the | + | particles could reach the Sun in the presence of a converging magnetic field. |
- | 1 we plot, as blue and red bands, an empirical parameter that estimates the number of | + | In Figure |
- | particles reaching the Sun | + | 1 we plot, as blue and red bands, an empirical parameter (dependent on this probability and the Alfvenic Mach number) that estimates the number of |
- | + | particles reaching the Sun. | |
- | determined by the local conditions at the front. | + | The bands are plotted for different critical Mach numbers (Mc) |
- | narrow first stage peak at 16 UT and the hours-long second stage of emission. The rise | + | determined by the local conditions at the shock front. |
- | in flux after 16:08 UT, shown in the inset, can be explained by the rapid change in shock | + | This normalized parameter reproduces both the |
+ | narrow first stage peak at 16:00 UT and the hours-long second stage of emission. | ||
+ | The rise in flux after 16:08 UT, shown in the inset, can be explained by the rapid change in shock | ||
geometry from quasi-perpendicular (Mc=2.7) to quasi-parallel (Mc=1.5). The apparent | geometry from quasi-perpendicular (Mc=2.7) to quasi-parallel (Mc=1.5). The apparent | ||
discontinuity in flux between ~16:30 and 18:00 UT is explained by the large increase in | discontinuity in flux between ~16:30 and 18:00 UT is explained by the large increase in | ||
Line 99: | Line 114: | ||
We have not attempted to take into account the effects of magnetic turbulence in this | We have not attempted to take into account the effects of magnetic turbulence in this | ||
- | study, but its effects | + | study, but its effects become apparent when we compare the model's broad spatial distribution |
- | of particle interactions on the visible disk with the | + | of particle interactions on the visible disk with the location of the |
- | centroid of the gamma radiation (Ref). This difference can be explained by | + | centroid of the γ radiation at the active region (Ref [4]). This difference can be explained by a large |
amount of turbulence, and therefore particle precipitation, near the active region | amount of turbulence, and therefore particle precipitation, near the active region | ||
relative to other locations on the visible disk. | relative to other locations on the visible disk. | ||
Line 108: | Line 123: | ||
==Comparison of Neutron Monitor Observations with Results of Shock Modeling== | ==Comparison of Neutron Monitor Observations with Results of Shock Modeling== | ||
- | We also investigated whether | + | We also investigated whether particle acceleration at the shock where it crosses field lines |
reaching Earth can explain the time history of relativistic protons observed early | reaching Earth can explain the time history of relativistic protons observed early | ||
in the GLE observed by the magnetically well connected Ft. Smith neutron monitor. The | in the GLE observed by the magnetically well connected Ft. Smith neutron monitor. The | ||
- | calculated time histories, corrected | + | calculated time histories, corrected with three potential proton transport times, are shown in Figure |
- | 4 | + | 4, along with two- and five-minute neutron monitor rates. The red curve shows |
the best fit to the neutron monitor data allowing for both shock and flare (using the | the best fit to the neutron monitor data allowing for both shock and flare (using the | ||
- | nuclear line time profile shown in Figure 2) particle components. Flare particles only | + | nuclear line time profile shown in Figure 2) particle components and a proton transport time of 12.5 minutes. Flare particles only |
contributed about 30% to the total rate for the first ten minutes of the event that is | contributed about 30% to the total rate for the first ten minutes of the event that is | ||
- | dominated by the shock component. The large | + | dominated by the shock component. The large proton transport time suggests a |
significant amount of interplanetary turbulence. | significant amount of interplanetary turbulence. | ||
- | |||
- | |||
==Conclusions== | ==Conclusions== | ||
- | Our analysis of the 2017 September 10 event provides | + | Our analysis of the 2017 September 10 event provides evidence for a common |
shock origin for protons producing the LPGRE and most of the particles observed in GLE72. | shock origin for protons producing the LPGRE and most of the particles observed in GLE72. | ||
+ | |||
+ | ==Acknowledgments== | ||
+ | We thank Alexis Rouillard, Illya Plotnikov, Ronald Murphy, Athanasios Papaioannou, and Yihong Wu who made significant contributions to this work. | ||
+ | |||
+ | == References == | ||
+ | |||
+ | [1] [http://adsabs.harvard.edu/abs/2018ApJ...869..182S/abstract "Characteristics of Late-phase >100 MeV Gamma-Ray Emission in Solar Eruptive Events"] | ||
+ | |||
+ | [2] [http://adsabs.harvard.edu/abs/1985ICRC....4..146F "Neutral Pion Production in Solar Flares"] | ||
+ | |||
+ | [3] [https://ui.adsabs.harvard.edu/abs/2020arXiv200400355K/abstract "Evidence for a Coronal Shock Wave Origin for Relativistic Protons Producing Solar Gamma-Rays and Observed by Neutron Monitors at Earth"] | ||
+ | |||
+ | [4] [https://ui.adsabs.harvard.edu/abs/2018ApJ...865L...7O/abstract "Fermi-LAT Observations of the 2017 September 10 Solar Flare"] | ||
+ | |||
+ | [5] [https://ui.adsabs.harvard.edu/abs/2017A%26A...608A..43P/abstract "The magnetic connectivity of coronal shocks from behind-the-limb flares to the visible solar surface during γ-ray events"] |
Latest revision as of 19:57, 8 April 2020
Nugget | |
---|---|
Number: | 375 |
1st Author: | Athanasios Kouloumvakos |
2nd Author: | Gerald Share |
Published: | 16 April 2020 |
Next Nugget: | Filament eruption |
Previous Nugget: | Using overlappogram data to find hot flare plasma |
List all |
Contents |
Introduction
The Fermi γ-ray observatory has shown that powerful solar eruptive events often emit copious fluxes of high-energy (>100 MeV) γ-rays, at times after the impulsive flare phase. These observations have become a powerful tool with which to explore the high-energy processes, such as particle acceleration, that constitute a major part of the eruptions' physical development. There have now been several Nuggets discussing what was earlier referred to as Long Duration Gamma-Ray Flares, including (No. 179, No. 227, No. 300, and recently No. 370, as well as No. 373)
Based on a detailed study of thirty >100 MeV γ-ray events observed by Fermi LAT, we decided that Late Phase Gamma-Ray Emission (LPGRE) best describes the characteristics of this phenomenon (Ref [1]):
- 1) the γ-ray emission is from pion decays;
- 2) it can begin close to the onset of the impulsive solar flare, but with a distinctly different time history, or up to two hours later;
- 3) rise times range from a few minutes to 6 hr and durations from 5 minutes to 20 hr;
- 4) there are typically ten times more >500 MeV protons producing the >100 MeV LPGRE than the flare >100 MeV γ-ray emission;
- 5) 28 of the 30 LPGRE events were associated with fast and broad Coronal Mass Ejections (CME's) (ejections CME) and 29 of the 30 were associated with Type ll radio emission;
- 6) LPGRE and >100 MeV solar energetic particle (SEP) durations appear to be correlated and the number of >500 MeV protons producing the LPGRE appear to range from 0.1 to 50% of the number in the accompanying SEP with large systematic effects; and
- 7) accompanying >100 keV impulsive bremsstrahlung suggests the presence of a suprathermal flare proton seed population that is accelerated by a shock wave and contributes to the LPGRE.
These characteristics support suggestions, beginning as early as the 1980's, that LPGRE is produced by the same CME-shock acceleration process that accelerates SEPs (Ref [2]). However, this premise is not universally accepted. In this Nugget we discuss a recent study (Ref [3]) of the 2017 September 10 solar eruptive event that produced high-energy γ-ray emission having a complex time history (Ref [4]) and a ground-level enhancement (GLE) designated as GLE72 (a numerical index) that began with SOL1956-02-23.
We examine a common shock-acceleration origin for both the LPGRE and GLE72. The >100 MeV γ-ray emission observed by the Large Area Telescope (LAT) on the Fermi satellite exhibits a weak impulsive phase, consistent with that observed in hard X-and γ-ray line flare emissions, and what appear to be two distinct stages of LPGRE. From a detailed modeling of the shock wave, we derive the 3D distribution and temporal evolution of various shock parameters, and we examine the shock wave magnetic connection with the visible solar disk. The evolution of shock parameters on field lines returning to the visible disk mirrors the two stages of LPGRE. The time history of shock parameters magnetically mapped to Earth agrees with the rates observed by the Fort Smith neutron monitor during the first hour of the GLE72 if we include a 30% contribution of flare-accelerated protons during the first ten minutes, having a release time following the time history of nuclear gamma-rays.
Comparison of Gamma-Ray Observations with Results of Shock Modeling
The black data points in Figure 1 show the time history of the >100 MeV γ-ray fluxes over the whole event and for the first 30 minutes (inset).
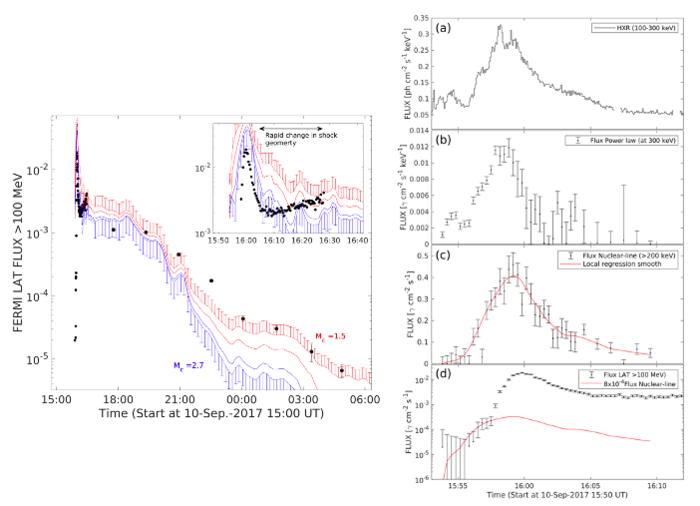
We compare the
time history of this high-energy emission for the first 15 minutes with lower energy
emissions in Figure 2. The 100-300 keV (RHESSI) plot in panel a) shows the impulsive
flare bremsstrahlung that extends to >1 MeV based on fits to the Fermi/GBM
spectra (panel b). These fits also reveal the presence of nuclear de-excitation γ-ray lines produced by
flare-accelerated ions (panel c). There is evidence for this flare component in the first
minutes of >100 MeV γ-ray emission before the rapid rise of the first stage of LPGRE at
~15:58 UT (panel d). The second stage of LPGRE becomes evident after 16:05 UT.
Observations at later times indicate that there was a striking decrease in the flux
between 16:30 and 18:00 UT.
We set out to determine whether what appear to be at least two stages of LPGRE can be
explained by CME related shock acceleration of protons onto magnetic field lines
returning to the visible solar disk. This study follows the CME mapping and shock
parameter procedures adapted in (Ref [5]). We used 3D modelling of the CME
expansion into the corona and interplanetery space to model various shock parameters
with time. In Figure 3a we plot the density compression ratio
along the shock front at 16:00 UT. This ratio is a measure of the efficiency for particle
acceleration. In Fig 3b we plot representative field lines connected to the shock front,
color coded with the density compression ratio. In Fig 3c we plot the ratio as projected
onto the solar disk by these field lines. At this time, the highest ratios, and therefore most of the particle acceleration,
appears to occur on closed field lines emanating from the active region with distant footpoints forming an ellipse. The pink shaded region is not
visible from Earth. Similar plots can be made for Alfvenic Mach number and magnetic field
direction relative to the shock normal.

Using this technique, we made maps of shock parameters on the visible solar disk as functions of time to construct their temporal evolution. In order to more accurately estimate the proton impact at the Sun, we took into account the probability that the particles could reach the Sun in the presence of a converging magnetic field. In Figure 1 we plot, as blue and red bands, an empirical parameter (dependent on this probability and the Alfvenic Mach number) that estimates the number of particles reaching the Sun. The bands are plotted for different critical Mach numbers (Mc) determined by the local conditions at the shock front. This normalized parameter reproduces both the narrow first stage peak at 16:00 UT and the hours-long second stage of emission. The rise in flux after 16:08 UT, shown in the inset, can be explained by the rapid change in shock geometry from quasi-perpendicular (Mc=2.7) to quasi-parallel (Mc=1.5). The apparent discontinuity in flux between ~16:30 and 18:00 UT is explained by the large increase in magnetic repulsion encountered by particles returning to the Sun from locations with low field strengths.
We have not attempted to take into account the effects of magnetic turbulence in this study, but its effects become apparent when we compare the model's broad spatial distribution of particle interactions on the visible disk with the location of the centroid of the γ radiation at the active region (Ref [4]). This difference can be explained by a large amount of turbulence, and therefore particle precipitation, near the active region relative to other locations on the visible disk.
Comparison of Neutron Monitor Observations with Results of Shock Modeling
We also investigated whether particle acceleration at the shock where it crosses field lines reaching Earth can explain the time history of relativistic protons observed early in the GLE observed by the magnetically well connected Ft. Smith neutron monitor. The calculated time histories, corrected with three potential proton transport times, are shown in Figure 4, along with two- and five-minute neutron monitor rates. The red curve shows the best fit to the neutron monitor data allowing for both shock and flare (using the nuclear line time profile shown in Figure 2) particle components and a proton transport time of 12.5 minutes. Flare particles only contributed about 30% to the total rate for the first ten minutes of the event that is dominated by the shock component. The large proton transport time suggests a significant amount of interplanetary turbulence.
Conclusions
Our analysis of the 2017 September 10 event provides evidence for a common shock origin for protons producing the LPGRE and most of the particles observed in GLE72.
Acknowledgments
We thank Alexis Rouillard, Illya Plotnikov, Ronald Murphy, Athanasios Papaioannou, and Yihong Wu who made significant contributions to this work.
References
[1] "Characteristics of Late-phase >100 MeV Gamma-Ray Emission in Solar Eruptive Events"
[2] "Neutral Pion Production in Solar Flares"
[4] "Fermi-LAT Observations of the 2017 September 10 Solar Flare"
RHESSI Nugget Date | 16 April 2020 + |
RHESSI Nugget First Author | Athanasios Kouloumvakos + |
RHESSI Nugget Index | 375 + |
RHESSI Nugget Second Author | Gerald Share + |