Flare Coronal Rain
From RHESSI Wiki
Line 69: | Line 69: | ||
This gives 1.88 s time resolution if one ignores niceties such as polarization and wavelength. | This gives 1.88 s time resolution if one ignores niceties such as polarization and wavelength. | ||
- | [[File:216f3.png| | + | [[File:216f3.png|500px|thumb|center|Fig. 2: Tracking the descent of the protuberance seen in the |
middle panel of Figure 2. | middle panel of Figure 2. | ||
Its steady motion towards smaller values of X corresponds essentially to the radial direction, | Its steady motion towards smaller values of X corresponds essentially to the radial direction, |
Revision as of 21:00, 19 December 2013
Nugget | |
---|---|
Number: | 216 |
1st Author: | Hugh Hudson |
2nd Author: | Säm Krucker |
Published: | December 23, 2013 |
Next Nugget: | TBD |
Previous Nugget: | The HEROES Mission: High Energy Replicated Optics to Explore the Sun |
List all |
Contents |
Introduction
The phenomenon of "coronal rain," a recent APOD topic, has has an extremely close identification with the physics of solar flares. More recently it has also become detectable in the quiet Sun, thanks to the greater sensitivity of modern high-resolution observations, but that is another interesting story. In this phenomenon, which had been noted as hydrogen H-alpha observations became commonplace in the early 20th century, material appears to condense as though magically out of the near-vacuum of the solar corona, and to cascade down along trajectories that certainly outline the orientation of the pervasive magnetic field there. Obviously matter is not being created, and a basic theoretical explanation was provided in 1965 by George B. Field (Ref. [1]). The magical appearance in H-alpha is just due to the recombination of hydrogen, which of course allows this line to emit, but only from the neutral state.
The state of the gas prior to its condensation into visible forms is now well understood, thanks to the development of radio and X-ray observational technique. A flare results when energy is released from the solar corona; this has the inevitable consequence of driving material up into the corona, and somewhat mysteriously heating it at the same time. In the most extreme cases this erupting materia escapes from the Sun in the form of a coronal mass ejection. A recent RHESSI Science Nugget describes some of the manifestations of the hot, over-dense loop-like structures that result from this cycling of mass between the lower and upper solar atmospheres.
HMI coronal observations
The Heliospheric and Magnetic Imager instrument (HMI) on board the Solar Dynamics Observatory is now providing a wholly new view of the last stages of development of the coronal mass cycle. As the loops cool, Field's thermal "instability" produces a bright enough excess emission to be visible even in the low corona by a simple telescope such as HMI - not optimized in any way, as a coronagraph would be, to see the normally faint corona. A recent paper (Ref. [2]) has revealed several features observed in this way by HMI, which is an excellent surprise to all concerned. HMI makes comprehensive spectroscopic measurements with full diagnosis of the polarization, a powerful aid in learning about the emission mechanisms involved.
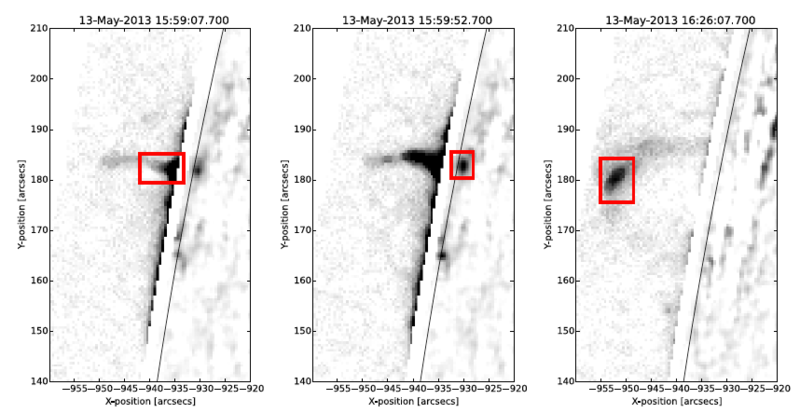
Rain, so what?
As the thermal instability progresses (see Ref. [3] for modern work on the dynamics of the hot plasmas), recombination happens, and suddenly low-excitation lines and continua can appear. We think that this is happening in some of the HMI coronal sources, in particular because we see downward motions in some of the ejecta. Figure 2 illustrates this for the source bracketed in the middle panel of Figure 1. To make this figure, we followed the outermost edge of the jet-like protuberance, using interleaved images from HMI's two cameras. This gives 1.88 s time resolution if one ignores niceties such as polarization and wavelength.
The inferred velocity in the projection of Fig. 2, based on a fit to the points, is 134 /s± 8 km/s. There is no perceptible acceleration. This is distinctly below the free-fall speed in the solar gravitational field, which is typical of coronal rain observed in other wayes, but the theory is incomplete - the basic physics may be understood, but reasonable modeling does not yet exist. HMI's design wavelength range brackets an Fe I line at 6173Å, but since the Fe has probably started off in its helium-like configuration (with two electrons), there may be substantial delays while two dozen more electrons turn up to finish the job of recombination. Thus we think that what HMI sees may have substantial contributions from other wavelengths, in particular continua. We identify three interesting and probably open problems:
(1) Why is the motion so slow, and why is there no acceleration? (2) What is actually emitting in the HMI passband? (3) What is left in the evacuated flux tube after the shower ends?
Conclusions
The observation of flare-associated coronal rain reported here opens a new window on this process, and one for which HMI can make an excellent contribution: it should see many cases, since it sees this one, and it will make polarization measurements of high quality as long as we stick to difference images.
References
- [1] Thermal Instability
- [2] Chromospheric and Coronal Observations of Solar Flares with the Helioseismic and Magnetic Imager
- [3] The Cooling of Coronal Plasmas. IV. Catastrophic Cooling of Loops
RHESSI Nugget Date | 23 December 2013 + |
RHESSI Nugget First Author | Hugh Hudson + |
RHESSI Nugget Index | 216 + |
RHESSI Nugget Second Author | Säm Krucker + |